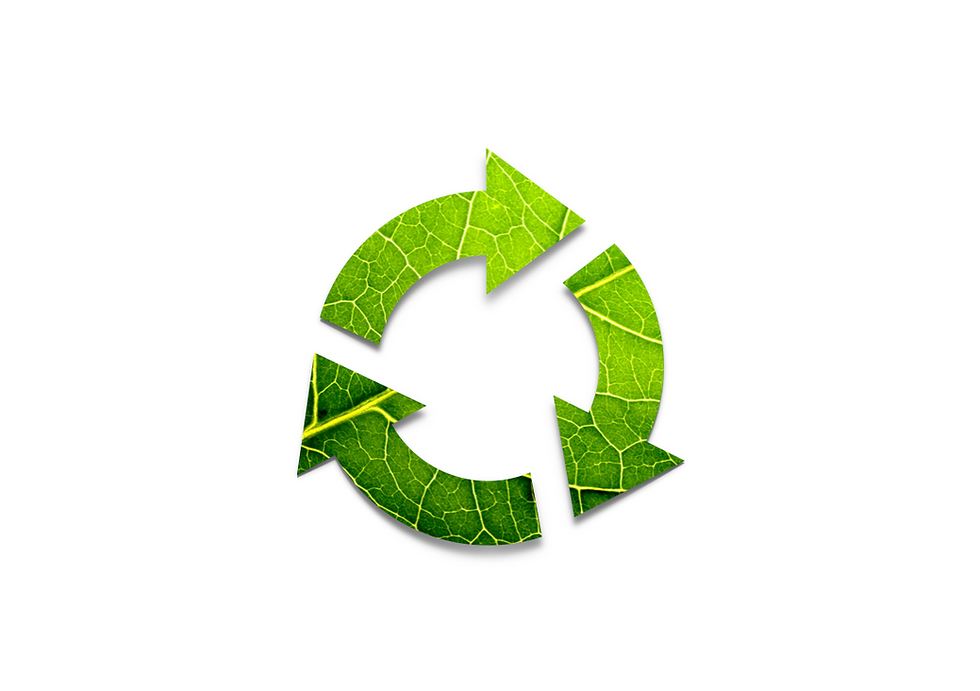
The biocomposite industry is emerging as a viable alternative to plastic in regards to the production of commercial products and fossil fuels. New biocomposite materials are steadily created as we approach an era that must address the consequences of previous decades of accumulated pollution. Fabricated from natural materials, biocomposites degrade in nature, reducing the toxic waste emitted by landfills. Therefore, interest in biocomposite production and utilisation is increasing worldwide in efforts to reduce the negative environmental impact effected by plastic waste. Though, will biocomposite materials eventually replace modern, petroleum-based plastics?
Pollution Crisis
Pollution is an increasingly concerning problem due to the escalating effects of global warming caused by air pollution. The industrial production sector is a major source of air pollutants, and industrial factories are among the most significant sources within this sector (Kampa & Castanas, 2008). Additionally, plastic effluents contaminating natural waters threaten surrounding wildlife.
As plastic became a commodity of modern living, the mass production of plastics has produced critical effects upon the environment. The production process emits contaminated fumes into the atmosphere and toxic leachates from landfills contaminate groundwater. These pollutants also negatively affect human health: the effects of contaminated fumes interspersed within the atmosphere “[range] from minor upper respiratory irritation to chronic respiratory and heart disease, lung cancer, acute respiratory infections in children and chronic bronchitis in adults, aggravating pre-existing heart and lung disease, [and] asthmatic attacks” (Kampa & Castanas, 2008). Likewise, residents within close proximity to landfills were noted to have an “[increased] risk of adverse health effects [such as] low birth weight, birth defects, [and] certain types of cancers” (Vrijheid, 2000). As landfills continue to be primary methods of waste disposal and factories a major source of pollutants, we should consider the materials we choose to consume and dispose of, particularly concerning their biodegradability and effect on the environment.
The adverse effects of plastics span to animal life and the ecosystem as well. A particular study by Eriksen et. al (2014) found an estimated 268,940 tons or 5.25 trillion floating plastic particles residing in our oceans, endangering marine wildlife and the Earth’s ecosystem. Effluents contaminating groundwater also spill into freshwaters, intoxicating fish and rodents with ammonia and inorganic minerals (Baderna et al., 2011). Given that the majority of plastics degrade at an extremely slow rate, we should continue examining our response to pollution in the longer term.
Without sufficient attention given to reducing air pollutants, the effects of global warming continue to intensify. The concentration of heavy metals in the atmosphere is particularly concerning “due to their recalcitrance and persistence in the environment” (Fu & Wang, 2010). Removing pollutants from the atmosphere is independently executed by the Earth’s flora, which is becoming progressively endangered as a result of deforestation and urbanisation. Furthermore, as the sole method of purifying the atmosphere becomes increasingly obsolete and as the population continues to grow, ramifications of pollution will become more prevalent in future generations.
Biocomposites
In response to deterring conditions, scientists and designers have generated composites from natural materials through the fortification of biofibers and matrix polymer systems (Mohanty et al., 2005). Due to their composition, they degrade in nature and leave no negative impact on the environment. Several new applications for the materials are created as the industry of biocomposites continues to grow.
Biocomposites exist in a variety of compositions ranging in molecular complexity. Because of their variance in characteristics, the potential applications for biocomposite materials are endless. Scientists continue to produce new composites and applications as the need for these ecologically sustainable materials increases.
Plastic was one of the first mass-produced products constructed from biocomposites. During the earlier half of the 18th century, plastic produced from the milk protein, casein, was used to create small items such as buttons and buckles. During this period, however, applications for the casein biocomposite were limited as creating such products required a tedious manufacturing process. In consequence, the industry became less popular and was eventually replaced by the oil-based plastics we use today (Brother, 1940).
QMILCH, a production and processing company based in Germany, re-initiated the production of casein products and expanded its potential applications. QMILK’s founder Anke Domaske, a fashion designer and microbiologist, formulated a composite from spoiled milk, aiming to reduce the environmental impact of chemically-produced fabrics. Resulting from this biocomposite was a plastic-like material— devoid of chemicals —that could be strung into fabrics for clothing. Domaske, beginning her career as a fashion designer, now uses this material to compliment her fashion designs sold worldwide to common consumers and famed individuals (QMILCH Deutschland GmbH).
Plastic food packagings are a common example of non-degradable waste that accumulates in landfills. As an alternative to plastic packaging, Universal Biopack’s director Suthep Vichakyothin shifted the company’s production of compact discs to bamboo and cassava biocomposite packaging material after realising its market potential and ecological sustainability. To construct their products, they use collected waste from bamboo chopsticks in addition to cassava starch, forming a biocomposite with the ability to dissolve in “room-temperature water within a week, leaving zero waste behind” (Karacs, 2017). The biocomposite is then cast into moulds according to the specifications of the client. Additionally, managing director of Universal Biopack Vara-Anong Vichakyothin advanced the production to Krathongs, ornaments used in Thailand’s light festivals, to combat increasing non-degrading styrofoam Krathongs.
Bamboo biocomposites are among the most valued in the industry due to their versatility and physical properties. A preference for bamboo biocomposites is rooted in their attractive characteristics: bamboo is “lightweight, nonabrasive, nontoxic, and biodegradable in nature” (Liu et al., 2012, 1469). Additionally, bamboo has proportionally high strength, a low production cost and is commonly utilised in “textile, paper, and construction” applications (Liu et al., 2012).
The discovery of growing fungi to develop materials of varying characteristics is a growing method amongst researchers and designers in the Netherlands.
Researchers Han Wosten and Maruizio Montalti from Utrecht University discovered that growing fungi in a mould and curing the organism to halt growth would result in a solid body:
“Fungi consist of many different filaments. Those filaments grow from a core and from some distance they start branching out, growing into a network of filaments. If you let the fungus grow into wood pulp, it would decompose the wood while at the same time glueing what is still left of the pulp together. […] If the growth is successful, you can remove the mould and make sure to stop the growing process of the microorganism, and this can be achieved by cooking the object. At that moment, the material becomes completely inert.”
Yet, production of fungus materials is not limited in textures and densities. Wosten and Montalti modified the fungi to generate a multitude of characteristics ranging from flexible, rubber-like materials to materials resembling plastic, leather and cork. Wosten and Montalti believe that materials composed of fungi will eventually replace the oil-based plastics we use today (Motherboard, 2015).
Designer Eric Klarenbeek is also advancing fungi biocomposite applications by means of constructing furniture from 3-D printed landfill waste. Klarenbeek uses pulverised natural waste, which eventually form fungi from bacteria, to fill 3-D printed objects. The fungus can be hardened to a specified degree through means of heating and inhibiting the fungi at a certain point during growth. With this method, Klarenbeek constructs protective casings for product shipments and an array of stylised furniture pieces. Like Wosten and Montalti, Klarenbeek aims to replace plastic products with environmentally sustainable fungus biocomposites (Motherboard, 2015).
Biocomposites in the Automotive Industry
Biocomposite utilisation in the automotive industry is a promising application in current times. Researchers are studying biofibres as an alternative to glass fibres for their exceptional properties, as previously stated: “low cost, low density, acceptable specific strength properties, ease of separation, carbon dioxide sequestration, and biodegradability” (Mohanty et al., 2002). At Michigan State University, researchers are working to create biocomposites from “hemp, kenaf, corn straw, and grass to replace plastic- and metal-based car components” that produce similar characteristics to materials currently employed in the industry (Mohanty et al., 2002). To automakers, biocomposite and biomaterial utilisation is a valued prospect for its benefits to the safety, weight and efficiency of vehicles. Automobile components composed of biofibrous materials exert equivalent performance at a lower weight while maintaining non-brittle characteristics during impacts. Fuel efficiency and passenger safety are therefore increased, creating an attractive product to the consumer and producer (Mohanty et al., 2002).
Biocomposite Sustainability in the Environment
Biocomposites are lauded for their advantage duality: they are ecologically sustainable and effective. They are seen as the future of plastics for their similar characteristics and contrasting ability to degrade in nature, reducing pollution in the atmosphere and waters. Biocomposite materials, sometimes referred to as bioplastics, are considered to be “a solution for [most] industries and society, which largely depended on materials made from plastic” (Marjadi, 2010). The production cost of biocomposite materials has deterred a number of manufacturers from utilising the composite, but a recent decrease in costs have been encouraging. Additionally, the cost of petroleum and petroleum-based products such as plastic has seen a consistent increase in price, which is countering the drawbacks of the production cost of biocomposite-based materials. On that account, researchers are confident in the eventual replacement of petroleum-based plastic with biocomposite materials.
Incineration, recycling and the conversion of waste are common approaches to waste management, however, these methods prove unsustainable in the longer term, producing negative effects on the environment. Considering the current methods of waste management, consumers should be conscious of the noxious effects of disposed consumables and their effluents on the environment.
Paper waste occupying landfills are subject to decomposition through saturation, converting into a pulp that releases toxic leachates into natural water sources. Additionally, the production of paper yields distinctive leachates through each manufacturing procedure of debarking, pulping, bleaching and washing harvested wood to create paper. As paper mills employ various production strategies and processes, ejected effluents will be dissimilar amongst these mills and thus require specialised applications for the decontamination of water sources. The difficulty of decontaminating waters from specific chemicals therefore prioritises a reduction in paper production as well as further knowledge of the “possible contaminants present in the wastewater, their origins and degree of toxicity and available treatment technologies” (Ali & Sreekrishnan, 2001).
Described by Peck and Daley (1994), “pulp mill effluent is a Pandora’s box of waste chemicals,” or an amalgamation of chemical compounds toxic to the environment. Several of these chemicals are recognised by the United States Environmental Protection Agency as “priority pollutants” and are known to be “recalcitrant to degradation and [persistent] in nature” (Ali & Sreekrishnan, 2001). With a thought to environmental sustainability, further discussions concerning waste management and prevention should be held to rectify the unsustainable practices of paper production and disposal.
Researchers have produced the method of pyrolysis to convert plastic waste into fuel, but the exhaust resulting from fuel combustion arguably counteracts the intent of waste reduction and repurposing by emitting pollutants into the atmosphere. Pyrolysis, a process in which “fuels, monomers, or other valuable materials [are produced] by thermal and catalytic cracking processes,” is seen as an effective method of repurposing accumulated plastic in landfills (Sheirs & Kaminsky, 2006). The reduction and conversion of plastics in landfills is effective for waste reduction but is counterproductive toward sustainability as it increases the concentration of greenhouse gases in the atmosphere and, therefore, the effects of global warming.
Biocomposite materials have presented a sustainable alternative to plastics with advantages of being light-weight, low cost, and biodegradable. The mass-production and implementation of biomaterials would significantly decrease the rate of amassing waste in landfills while preserving fossil-based raw materials and reducing carbon dioxide emissions (Mohanty, Misra, & Hinrichsen, 2000). Furthermore, research on biocomposite applications and experiments with biocomposite properties provide increasing prospects for ecological sustainability (Davis & Song, 2006). Provided the levelled performance of biocomposites to plastics as well as their biodegradable nature, biocomposites are emerging as a sustainable material source for utilisation in mass-production.
Biocomposite Applications and Projections
As potential applications for biocomposite materials increase, the biocomposite industry continues to grow. Biocomposite materials are in considerable demand from the construction and automotive industries, with characteristics most advantageous to applications within these industries. Appropriately, market projections for the biocomposite industry predict a significant growth within the next decade (Ariadurai, 2013).
Applications in the construction industry favour biomaterials for their lightweight, moisture resistant, and low maintenance attributes. Current applications in construction for biocomposite materials include utilisation in “decking, railings, balusters, fences,” and several additional applications (Markets and Markets, 2017). Taking advantage of the biocomposites’ superior strength properties, they are further used in cement matrices to make “panels, claddings, roofing sheets and tiles, slabs and beams” (Ariadurai, 2013). The utilisation of biocomposite materials in the construction industry continues to grow the biocomposite market as new applications for the composite are produced.
Biocomposite materials in the automotive industry have also experienced increased applications for their impact-resistant, light-weight, and non-toxic properties (Markets and Markets, 2017). Automotive manufacturers seeking light-weight, low-cost, thermally insulated, easily produced materials seek biocomposites for their versatile strengths and utilise the composite to produce components such as “door panels, inserts, rear parcel shelves, setbacks, spare tire covers and other interior trims” (Ariadurai, 2013). Biocomposite applications in the automotive industry are viewed as the strongest source of market growth as its features are predominantly attuned to the needs of automotive manufacturers.
With consideration to the growing applications of biocomposite materials, several studies on the biocomposite market have predicted growth of the industry in the next decade. A study conducted by Duncan et al. (2008) predicted the industry to grow “3-6 percent per year through 2025, with bio-based chemicals’ share of that market rising from 2 percent currently to 22 percent or more by 2025." Another study executed by Future Market Insights (2018) estimated the biocomposite market “to reach $450 billion by 2025,” and in the event that biocomposite-based materials dominate material resources, the chemical industry will expand to over $2 trillion and provide more than $500 billion in revenue per year (Duncan et al., 2008). Finally, in 2005, research was published by Wolf et al. analysing the probability of biocomposite-based plastics mitigating atmospheric pollution. They found that by 2025, “bio-based polymers will be able to meaningfully compensate for the environmental impacts of the economy as a whole." Based on the aforementioned market studies, a significant growth in the biocomposite market is projected within the next decade.
Biocomposites are a viable alternative to petroleum-based plastics with advantages of mitigating pollution and leaving minimal impact on the environment. The profitable properties and versatile applications of biocomposite materials leave them in high demand by several industries, of which the automotive and construction industries are highest to demand. The market for biodegradable and environmentally sustainable biocomposites is therefore expected to make significant growth in the coming years. Given the collective information, biocomposite-based materials and their applications are predicted to eventually replace dominating materials and increase in production.
Comments